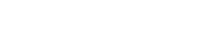
Does Timescapes Disprove Dark Energy?
Season 10 Episode 32 | 14m 30sVideo has Closed Captions
Maybe dark energy doesn't exist?
The universe is expanding and that expansion is accelerating under the power of dark energy and eventually all matter and energy will be dispersed over such unthinkable distances that nothing can stop space from blowing up infinitely. Unless of course cosmologists blundered and dark energy doesn't even exist. Then it's back to the drawing board.
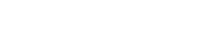
Does Timescapes Disprove Dark Energy?
Season 10 Episode 32 | 14m 30sVideo has Closed Captions
The universe is expanding and that expansion is accelerating under the power of dark energy and eventually all matter and energy will be dispersed over such unthinkable distances that nothing can stop space from blowing up infinitely. Unless of course cosmologists blundered and dark energy doesn't even exist. Then it's back to the drawing board.
How to Watch PBS Space Time
PBS Space Time is available to stream on pbs.org and the free PBS App, available on iPhone, Apple TV, Android TV, Android smartphones, Amazon Fire TV, Amazon Fire Tablet, Roku, Samsung Smart TV, and Vizio.
Providing Support for PBS.org
Learn Moreabout PBS online sponsorshipThe universe is expanding and that expansion is accelerating under the power of dark energy and eventually all matter and energy will be dispersed over such unthinkable distances that nothing can stop space from blowing up infinitely.
Unless of course cosmologists blundered and dark energy doesn't even exist.
Then it's back to the drawing board.
Hey everyone, quick update before we start!
We've observed that the YouTube algorithm isn’t promoting our episodes within the community as much, so we’re conducting an experiment.
Over the next few episodes, we’ll test whether increasing likes and comments impacts sharing.
If you'd like to be a data point in this experiment, hit like and/or leave a comment.
And if you’re new and enjoying this, subscribe and please press the bell.
We’ll analyze the results and report back after a few episodes!
A paper came out in December claiming that dark energy—widely held to be the dominant form of energy in the universe, and one the the central components of our modern cosmological model—simply doesn’t exist.
The paper, by Antonia Seifert and team at the University of Canterbury in New Zealand, claims that the apparent accelerating expansion comes from an oversimplification of our model of the expanding universe.
The researchers state that the current Lambda-CDM model doesn’t properly take into account the fact that time flows differently in different parts of the universe.
In particular, time flows slower in regions of strong gravity—so in the dense clusters and filaments of the cosmic web.
And it flows faster in the vast, empty regions in between.
This results in a landscape of shifting time flow—in which some parts of the universe may be billions of years older than others.
So, instead of a landscape, more of a timescape.
The timescape model was first proposed by David Wiltshire in 2007, and in this picture, faster-ticking parts of the universe will have expanded more than slower-time parts, and this effect can exactly mimic the apparent accelerating expansion, but without any acceleration at all at least according to this team.
Now, people have been saying that dark energy doesn’t exist since its discovery, with varying levels of quackery.
This particular proposal got more attention than usual, in part because it’s definitely not quackery.
The proposal is scientifically well-founded and the researchers are serious people.
There’s also a certain elegance to it that I hope I can convey to you.
None of this means the idea is right, and I’ll also try to convey why it’s probably not true.
First the super-quick review on dark energy.
The universe is expanding, and we’ve measured the history of that expansion.
One of the most important ways we do that is by measuring the distances to Type 1-a supernovae.
These exploding white dwarf stars pop with predictable brightnesses.
Light from these explosions travels to us over great distances, and two things happen on that journey.
One is that the light is dimmed as photons spread out through the universe.
This tells us how far and for how much time the light traveled.
Two is that those same photons are stretched—redshifted—by the expansion of the universe as they travel.
This tells us how much expansion happened during their travel time.
Put these together for many supernovae back through cosmic time and we get an expansion history for the universe.
The results of the first big supernova surveys came in in the late 90s, and scientists had expected to find that the expansion rate was slowing down due to gravity.
It had to be because Einstein’s general theory of relativity only allowed an inward-pulling gravitational force.
To everyone’s great surprise, these studies found that the expansion was accelerating.
At first glance this made no sense under the then-current version of general relativity.
However, adding an additional piece to GR—the cosmological constant—provided exactly the acceleration needed to explain the observations.
We don’t really know what causes this accelerating expansion—top contender is some sort of energy of the vacuum itself.
But whatever it is, we call it dark energy and its discovery scored our supernova collectors the Nobel prize in physics.
The existence of dark energy in the form of a cosmological constant is now part of our dominant mathematical description of how the universe works on the largest scales— the Lambda-CDM model.
Lambda-CDM does such a good job of explaining a whole range of observations that it’s hard to avoid the conclusion that something like dark energy must exist.
On the other hand, we’ve never really measured cosmic acceleration directly.
We’ve just found that models with dark energy fit the data better than models without it.
But what if there’s some other founding assumption behind our model of cosmic expansion that is wrong?
That’s basically what the timescape model is saying.
To understand the different factors driving the expansion of the universe, we need to solve the equations of general relativity for the entire universe.
That’s only possible with some pretty major simplifications.
The main one is that we assume that the matter in the universe is spread out smoothly everywhere—it’s both homogeneous and isotropic—no large-scale lumps or directionality.
That lets us describe the effect of matter with a single number—the matter density—rather than having to solve for every chunk of matter separately.
The result is the Friedmann equations, which we’ve picked apart before, and they describe how the size of the universe changes over time, driven by smoothly distributed matter and constant dark energy.
Add the appropriate amounts of both and you can describe our universe—and that’s the Lambda-CDM model.
Although the approximation of smoothness seems to be very good on large scales, we know that the universe is not smooth on smaller scales.
At distances smaller than a billion light years or so, this smooth matter has fragmented into enormous galaxy clusters and the filaments of matter connecting them.
And bound by this cosmic web are giant empty patches—cosmic voids.
These structures do have an effect on the photons passing through them.
For example, photons gain some energy falling into deep gravitational fields, resulting in blue-shift, and lose a little energy climbing out of them, resulting in redshift.
So a supernova that explodes somewhere deep in the Laniakea supercluster might gain some redshift from traveling to us through the expanding space, and more redshift from the fact that it has to fight against the supercluster’s gravitational field to reach the Milky Way, out on Laniakea’s periphery.
Local gravitational fields also affect the speeds of nearby matter, and so while that supernova is mostly moving away due to cosmic expansion, it might have some extra velocity either towards or away due to its home galaxy moving within Laniakea’s gravitational field.
We have to take care to account for this stuff, particularly for supernovae in the relatively nearby universe.
In fact, we talk about the possible effect of local structure in a previous video.
But if the supernova happened more than a few hundred million light years away then its photons will pass through multiple voids and superclusters on their way to us.
All that blue-shifting and redshifting will cancel out.
If that’s the case, then the Friedmann equations and Lambda-CDM should do a perfectly good job at describing the expanding universe experienced for those photons.
At least, that’s what most people think.
The timescape model says otherwise.
Like I said at the start of this video, time flows slower in regions of high gravity and faster in low gravity—this gravitational time dilation is a known and well tested feature of general relativity.
But the timescape model proposed that differences in time flow should have another effect—it should change the rate of expansion of the universe from one place to another.
It’s straightforward enough.
The more time that passes the bigger the universe gets, so if more time has passed in a cosmic void compared to a region of higher density, that void will have expanded more.
This differential expansion has an effect on photons traveling through these regions.
For example, photons are stretched out or redshifted more as they travel through the faster-expanding voids compared to dense regions.
As matter becomes more and more clumped together over time, the fraction of the universe with voids grows.
This means that photons traveling through the late universe spend more time in voids and so pick up more of this extra redshift.
In the Lambda-CDM model, it’s the accelerating expansion of the universe that gives photons their extra redshift in later times.
But in the timescape model, it’s the increasing void fraction, and, supposedly, no global acceleration is needed in timescape.
The timescape model has been around since 2007 and so the reason for the recent buzz is that the team just published their analysis of the results of the biggest current supernova survey—Pantheon+.
In general they find that timescape gives a better fit to the supernova data than Lambda-CDM.
You ca n see it on this plot, which shows the Bayes factor—basically the ratio of how good timescape is compared to Lambda-CDM.
If we’re in the blue region then timescape is preferred.
The x-axis is the redshift cut.
Points on the left use all the supernovae, including the nearby ones.
As we go to right we cut out supernovae starting from the closest to the more distant, so that over here we’re only including the most distant ones.
This is a nice way to show the result because the nearby supernovae should be more affected by inhomogeneities—more influenced by the local lumpiness of the universe.
So it makes some sense that Lambda-CDM doesn’t do as well there, with its assumption of perfect smoothness.
Further away, that lumpiness averages out and timescape and Lambda-CDM provide similar results, although according to this, timescape still does a bit better at all distances.
So, is this right?
Has dark energy been disproved?
Well, if the only evidence for dark energy were these supernova results, then this might be bad news for dark energy and the lambda-CDM model.
That’s because, in some sense, the timescape model is simpler than Lambda-CDM.
It only uses raw general relativity, just as Einstein conceived it, with no need for the addition of a cosmological constant.
It uses the base theory plus the effect of structures that we know exist in the universe.
Occam’s razor tells us that if two hypotheses yield identical results you should favor the simpler hypothesis.
By that logic timescape seems to win.
The amount of time that lambda-CDM has been around should not, by itself, make us favor the older theory.
After all, Ptolemy’s Earth-centered model of the solar system was around for a millennium and a half before Copernicus came along with his simpler Sun-centered model.
But the test of time is only relevant if it actually involves additional tests.
And indeed there are additional tests for Lambda-CDM.
This model provides a good description of more than just the supernova results.
For example, baryon acoustic oscillations—reverberating sound waves from very early times—are now frozen as faint rings in the distribution of galaxies on the sky.
The size of these rings gives an independent way to measure the expansion rate that is not affected by how cosmic voids redshift photons.
And the BAO observations also strongly indicate accelerating expansion and support Lambda-CDM.
There’s also the evolution of large scale structure, which nicely match the simulations we run under Lambda-CDM.
And there’s the fact that the universe is geometrically very flat, and for that to be the case we need 70% of the energy in the universe to be dark energy.
It may be that the timescape model can explain these too, but so far it hasn’t.
Another major issue with the timescape model is that it requires quite large differences in timeflow between the cosmic voids and the dense regions of the universe—large enough that billions of years more would have passed in the voids compared to the superclusters.
Such large differences are not the consensus.
For example, Ethan Seigel points out that the relative flow of time between these regions should result in an age differential of only 100s to 1000s of years, which isn’t anywhere near enough to produce the effect that timescape claims.
I should also point out that the researchers themselves admit that more work needs to be done.
For example, supernova distances are not at all easy to figure out, and require many steps of calibration.
The timescape team acknowledges that some of this requires further work.
So, yeah, dark energy didn’t go away.
Lambda-CDM still gives us the most consistent description of the large-scale universe, across multiple independent observations.
At the same time, Lambda-CDM has its challenges—there’s the now-famous Hubble tension—a mismatch between the expansion rate measured from supernovae versus the rate inferred under Lambda-CDM from observations of the cosmic microwave background.
And then there’s the recent Dark Energy Survey Instrument result that the cosmological constant may be actually changing over time.
It’s not clear that timescape can sort out these issues, but any chink in the armour of Lambda-CDM is a good motivation for exploring other models.
And maybe part of the solution will for us to be more careful in how we model the temporal landscape of spacetime.